General Information/ Impact of IS on Genome Evolution - The Importance of Time Scale
ISs have had an important impact on genome structure and function. Several of these effects are considered in the following sections. In this context it is useful to understand the time scales involved in these processes since they are often confounded. Evolutionary time is used to compare species (106 years), historical time in comparisons within or between populations (102 - 104 years), variety time in selection experiments (1 - 102 years), and laboratory time for ongoing events such as experimental measurement of transposition frequencies or in biochemical analyses (10-3 - 1 years) (Alan Schulman, pers comm.). Thus a “burst” of transposition in evolutionary time is many orders of magnitude longer than a “burst” of transposition in experimental biology.
IS expansion, elimination and genome streamlining
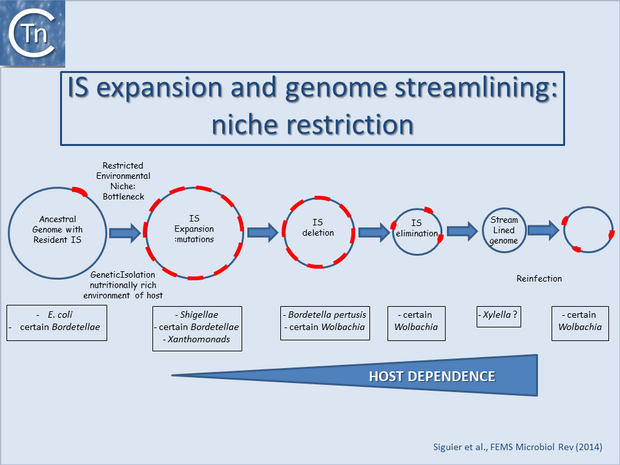
IS can undergo massive expansion and loss accompanied by gene inactivation and decay, genome rearrangement and genome reduction. Clearly, host lifestyle strongly influences these IS-mediated effects on genome structure, presumably by determining the level of genetic isolation of the microbial population. Factors affecting this include: whether the bacteria are ectosymbionts, primary endosymbionts having long evolutionary histories with their hosts, or secondary endosymbionts with more recent associations; whether they are transmitted in a strictly vertical manner or pass through a step of horizontal transfer via reinfection or passage through a second host vector[1][2][3][4].
IS expansion has been commonly observed in bacteria with recently adopted fastidious, host-restricted lifestyles. Those which may have more ancient host-restricted lifestyles (e.g. Wigglesworthia in the Tsetse fly; Buchnera aphidicola in the aphid; Blochmannia floridanus in the ant) tend to possess small streamlined genomes with few pseudogenes or MGEs (see [5][3]).
One view is that IS expansion is an early step in this genome reduction process[6][7][8][9] (Fig.15.1; [10][11]). This results from a decrease in strength and efficacy of purifying selection due to the shift from free to intracellular lifestyles[7]. It is reinforced by a phenomenon known as Muller’s ratchet which leads to the irreversible accumulation of mutations in a confined intracellular environment[12][13][14]. In the nutritionally rich environment of the host, many genes of free-living bacteria are inessential. Enhanced genetic drift would allow fixation of slightly deleterious mutations in the population, facilitated by the occurrence of successive population bottlenecks. The more genetically isolated the bacterial population, the more acute would be the effect. Indeed, many examples of this can be found among intracellular endosymbionts. This initial stage of transition from free-living to host-dependence would therefore result in an accumulation of pseudogenes which will eventually be eliminated by so-called deletional bias[15][16]. Clearly, the activities of MGEs, and of ISs in particular, make them important instruments in these processes. IS expansion would contribute to pseudogenisation by IS-mediated intrachromosomal recombination and genome reduction[13][15][17][18] by their capacities to generate deletions (see [19]). Such deletions would also eventually lead to complete or partial elimination of the ISs themselves. These processes are shown schematically in Fig.15.1.
There are many striking examples of IS expansions in bacterial genomes. The first to be identified was Shigella from the pre-genomics era[20][21]. But IS expansion identified from sequenced genomes has been implicated in generating the present day Bordetella pertussis and B. parapertusis, Yersinia pestis, Enterococcus faecium, Mycobacterium ulcerans and many others. In at least some of these cases it has been argued that large scale genome rearrangements and deletions associated with IS expansion have improved the ability of the bacterium to combat host defenses for example by changing surface antigens and regulatory circuitry. This has been particularly well documented in the Bordetellae[22][23].
The phenomenon is also common among endosymbionts such as Wolbachia sp. These are considered ancient endosymbionts which might be expected to possess more streamlined genomes. However, evidence has been presented that they have been subjected to several waves of invasion and elimination of ISs[24]. This may be related to the fact that they are not strictly transmitted vertically but may also undergo relatively low levels of horizontal transmission and coinfection. Other symbionts or host-restricted bacteria also contain high IS loads. These include organisms such as Orientia tsutsugamushi, various Rickettsia, Sodalis glossinidius, Amoebophilus asiaticus 5a2, the symbiont of the marine oligochaete Olavius algarvensis, the Bacteroidete Cardinium hertigii, a symbiont of the parasitic wasp Encarsia pergandiella, and the primary symbionts of grain weevils. These obligate intracellular bacteria may carry intercellular MGEs such as phage[25][26] and conjugative elements[27] capable of acting as IS vectors and motors of horizontal gene transfer. Similar arguments might be used for other niche-restricted prokaryotes to explain increased IS loads found in some extremophiles (e.g. Sulfolobus solfataricus and certain cyanobacteria)[28][29][30][31].
Although IS expansion is generally assumed to occur stochastically over periods of evolutionary time, it has recently been observed that the Olavius algarvensis symbionts express significant levels of transposase[32]. This raises the possibility that transposase expression is deregulated in this symbiont system. However, another symbiont, Amoebophilus asiaticus, with a high IS load, shows no evidence of recent transposition activity in spite of extensive IS transcription[33]. In view of the time scales involved, only a very small but sustained increase in transposition activity might be needed to give rise to the high loads observed. Further exploration of the relationship between IS gene expression and transposition activity is clearly essential to understanding the dynamics of ISs in these and other systems.
Of course, different ISs are involved in different expansions, and it is therefore important to understand IS diversity and properties. This is clearly evident in studies concerning the behavior of IS on storage of bacterial strains, where certain IS appear more active than others[34][35].Their detailed effects on the host genome will depend on their particular transposition mechanisms. For example, IS target specificity will have profound effects on the way the host genome is shaped.
Bibliography
- ↑ Bordenstein SR, Reznikoff WS . Mobile DNA in obligate intracellular bacteria. - Nat Rev Microbiol: 2005 Sep, 3(9);688-99 [PubMed:16138097] [DOI]
- ↑ Bordenstein SR, Paraskevopoulos C, Dunning Hotopp JC, Sapountzis P, Lo N, Bandi C, Tettelin H, Werren JH, Bourtzis K . Parasitism and mutualism in Wolbachia: what the phylogenomic trees can and cannot say. - Mol Biol Evol: 2009 Jan, 26(1);231-41 [PubMed:18974066] [DOI]
- ↑ 3.0 3.1 Moya A, Peretó J, Gil R, Latorre A . Learning how to live together: genomic insights into prokaryote-animal symbioses. - Nat Rev Genet: 2008 Mar, 9(3);218-29 [PubMed:18268509] [DOI]
- ↑ Silva FJ, Latorre A, Moya A . Genome size reduction through multiple events of gene disintegration in Buchnera APS. - Trends Genet: 2001 Nov, 17(11);615-8 [PubMed:11672844] [DOI]
- ↑ Bordenstein SR, Reznikoff WS . Mobile DNA in obligate intracellular bacteria. - Nat Rev Microbiol: 2005 Sep, 3(9);688-99 [PubMed:16138097] [DOI]
- ↑ Plague GR, Dunbar HE, Tran PL, Moran NA . Extensive proliferation of transposable elements in heritable bacterial symbionts. - J Bacteriol: 2008 Jan, 190(2);777-9 [PubMed:17981967] [DOI]
- ↑ 7.0 7.1 Moran NA, Plague GR . Genomic changes following host restriction in bacteria. - Curr Opin Genet Dev: 2004 Dec, 14(6);627-33 [PubMed:15531157] [DOI]
- ↑ Touchon M, Rocha EP . Causes of insertion sequences abundance in prokaryotic genomes. - Mol Biol Evol: 2007 Apr, 24(4);969-81 [PubMed:17251179] [DOI]
- ↑ Gil R, Belda E, Gosalbes MJ, Delaye L, Vallier A, Vincent-Monégat C, Heddi A, Silva FJ, Moya A, Latorre A . Massive presence of insertion sequences in the genome of SOPE, the primary endosymbiont of the rice weevil Sitophilus oryzae. - Int Microbiol: 2008 Mar, 11(1);41-8 [PubMed:18683631]
- ↑ Siguier P, Gourbeyre E, Chandler M . Bacterial insertion sequences: their genomic impact and diversity. - FEMS Microbiol Rev: 2014 Sep, 38(5);865-91 [PubMed:24499397] [DOI]
- ↑ Siguier P, Gourbeyre E, Varani A, Ton-Hoang B, Chandler M . Everyman's Guide to Bacterial Insertion Sequences. - Microbiol Spectr: 2015 Apr, 3(2);MDNA3-0030-2014 [PubMed:26104715] [DOI]
- ↑ Moran NA . Accelerated evolution and Muller's rachet in endosymbiotic bacteria. - Proc Natl Acad Sci U S A: 1996 Apr 2, 93(7);2873-8 [PubMed:8610134] [DOI]
- ↑ 13.0 13.1 Andersson SG, Kurland CG . Reductive evolution of resident genomes. - Trends Microbiol: 1998 Jul, 6(7);263-8 [PubMed:9717214] [DOI]
- ↑ Silva FJ, Latorre A, Moya A . Why are the genomes of endosymbiotic bacteria so stable? - Trends Genet: 2003 Apr, 19(4);176-80 [PubMed:12683967] [DOI]
- ↑ 15.0 15.1 Mira A, Ochman H, Moran NA . Deletional bias and the evolution of bacterial genomes. - Trends Genet: 2001 Oct, 17(10);589-96 [PubMed:11585665] [DOI]
- ↑ Moran NA, Mira A . The process of genome shrinkage in the obligate symbiont Buchnera aphidicola. - Genome Biol: 2001, 2(12);RESEARCH0054 [PubMed:11790257] [DOI]
- ↑ Andersson JO, Andersson SG . Insights into the evolutionary process of genome degradation. - Curr Opin Genet Dev: 1999 Dec, 9(6);664-71 [PubMed:10607609] [DOI]
- ↑ Lawrence JG, Hendrix RW, Casjens S . Where are the pseudogenes in bacterial genomes? - Trends Microbiol: 2001 Nov, 9(11);535-40 [PubMed:11825713] [DOI]
- ↑ Mahillon J, Chandler M . Insertion sequences. - Microbiol Mol Biol Rev: 1998 Sep, 62(3);725-74 [PubMed:9729608] [DOI]
- ↑ Nyman K, Nakamura K, Ohtsubo H, Ohtsubo E . Distribution of the insertion sequence IS1 in gram-negative bacteria. - Nature: 1981 Feb 12, 289(5798);609-12 [PubMed:6258088] [DOI]
- ↑ Ohtsubo H, Nyman K, Doroszkiewicz W, Ohtsubo E . Multiple copies of iso-insertion sequences of IS1 in Shigella dysenteriae chromosome. - Nature: 1981 Aug 13, 292(5824);640-3 [PubMed:6265806] [DOI]
- ↑ Parkhill J, Sebaihia M, Preston A, Murphy LD, Thomson N, Harris DE, Holden MT, Churcher CM, Bentley SD, Mungall KL, Cerdeño-Tárraga AM, Temple L, James K, Harris B, Quail MA, Achtman M, Atkin R, Baker S, Basham D, Bason N, Cherevach I, Chillingworth T, Collins M, Cronin A, Davis P, Doggett J, Feltwell T, Goble A, Hamlin N, Hauser H, Holroyd S, Jagels K, Leather S, Moule S, Norberczak H, O'Neil S, Ormond D, Price C, Rabbinowitsch E, Rutter S, Sanders M, Saunders D, Seeger K, Sharp S, Simmonds M, Skelton J, Squares R, Squares S, Stevens K, Unwin L, Whitehead S, Barrell BG, Maskell DJ . Comparative analysis of the genome sequences of Bordetella pertussis, Bordetella parapertussis and Bordetella bronchiseptica. - Nat Genet: 2003 Sep, 35(1);32-40 [PubMed:12910271] [DOI]
- ↑ Preston A, Parkhill J, Maskell DJ . The bordetellae: lessons from genomics. - Nat Rev Microbiol: 2004 May, 2(5);379-90 [PubMed:15100691] [DOI]
- ↑ Cerveau N, Leclercq S, Leroy E, Bouchon D, Cordaux R . Short- and long-term evolutionary dynamics of bacterial insertion sequences: insights from Wolbachia endosymbionts. - Genome Biol Evol: 2011, 3;1175-86 [PubMed:21940637] [DOI]
- ↑ Hsia R, Ohayon H, Gounon P, Dautry-Varsat A, Bavoil PM . Phage infection of the obligate intracellular bacterium, Chlamydia psittaci strain guinea pig inclusion conjunctivitis. - Microbes Infect: 2000 Jun, 2(7);761-72 [PubMed:10955956] [DOI]
- ↑ Read TD, Myers GS, Brunham RC, Nelson WC, Paulsen IT, Heidelberg J, Holtzapple E, Khouri H, Federova NB, Carty HA, Umayam LA, Haft DH, Peterson J, Beanan MJ, White O, Salzberg SL, Hsia RC, McClarty G, Rank RG, Bavoil PM, Fraser CM . Genome sequence of Chlamydophila caviae (Chlamydia psittaci GPIC): examining the role of niche-specific genes in the evolution of the Chlamydiaceae. - Nucleic Acids Res: 2003 Apr 15, 31(8);2134-47 [PubMed:12682364] [DOI]
- ↑ Blanc G, Ogata H, Robert C, Audic S, Claverie JM, Raoult D . Lateral gene transfer between obligate intracellular bacteria: evidence from the Rickettsia massiliae genome. - Genome Res: 2007 Nov, 17(11);1657-64 [PubMed:17916642] [DOI]
- ↑ Papke RT, Ramsing NB, Bateson MM, Ward DM . Geographical isolation in hot spring cyanobacteria. - Environ Microbiol: 2003 Aug, 5(8);650-9 [PubMed:12871232] [DOI]
- ↑ Brügger K, Torarinsson E, Redder P, Chen L, Garrett RA . Shuffling of Sulfolobus genomes by autonomous and non-autonomous mobile elements. - Biochem Soc Trans: 2004 Apr, 32(Pt 2);179-83 [PubMed:15046567] [DOI]
- ↑ Allewalt JP, Bateson MM, Revsbech NP, Slack K, Ward DM . Effect of temperature and light on growth of and photosynthesis by Synechococcus isolates typical of those predominating in the octopus spring microbial mat community of Yellowstone National Park. - Appl Environ Microbiol: 2006 Jan, 72(1);544-50 [PubMed:16391090] [DOI]
- ↑ Filée J, Siguier P, Chandler M . Insertion sequence diversity in archaea. - Microbiol Mol Biol Rev: 2007 Mar, 71(1);121-57 [PubMed:17347521] [DOI]
- ↑ Kleiner M, Young JC, Shah M, VerBerkmoes NC, Dubilier N . Metaproteomics reveals abundant transposase expression in mutualistic endosymbionts. - mBio: 2013 Jun 18, 4(3);e00223-13 [PubMed:23781067] [DOI]
- ↑ Schmitz-Esser S, Penz T, Spang A, Horn M . A bacterial genome in transition--an exceptional enrichment of IS elements but lack of evidence for recent transposition in the symbiont Amoebophilus asiaticus. - BMC Evol Biol: 2011 Sep 26, 11;270 [PubMed:21943072] [DOI]
- ↑ Naas T, Blot M, Fitch WM, Arber W . Insertion sequence-related genetic variation in resting Escherichia coli K-12. - Genetics: 1994 Mar, 136(3);721-30 [PubMed:7911771] [DOI]
- ↑ Naas T, Blot M, Fitch WM, Arber W . Dynamics of IS-related genetic rearrangements in resting Escherichia coli K-12. - Mol Biol Evol: 1995 Mar, 12(2);198-207 [PubMed:7700149] [DOI]